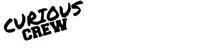
Speed of Sound
5/27/2022 | 26m 46sVideo has Closed Captions
Speedy sounds with air drums, funnel fun, and blaring bottles!
Speedy sounds with air drums, funnel fun, and blaring bottles! The Crew is all ears as they “pump up the volume” and explore the speed of sound with Dr. Rob! STEM Challenge: Making an auditory loop Curious About Careers: Sonic Strategist, Kristen Lueck
Problems with Closed Captions? Closed Captioning Feedback
Problems with Closed Captions? Closed Captioning Feedback
Curious Crew is a local public television program presented by WKAR
Support for Curious Crew Season 10 is provided by
MSU Federal Credit Union
Consumers Energy Foundation
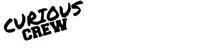
Speed of Sound
5/27/2022 | 26m 46sVideo has Closed Captions
Speedy sounds with air drums, funnel fun, and blaring bottles! The Crew is all ears as they “pump up the volume” and explore the speed of sound with Dr. Rob! STEM Challenge: Making an auditory loop Curious About Careers: Sonic Strategist, Kristen Lueck
Problems with Closed Captions? Closed Captioning Feedback
How to Watch Curious Crew
Curious Crew is available to stream on pbs.org and the free PBS App, available on iPhone, Apple TV, Android TV, Android smartphones, Amazon Fire TV, Amazon Fire Tablet, Roku, Samsung Smart TV, and Vizio.
Providing Support for PBS.org
Learn Moreabout PBS online sponsorship- [Rob] Are you feeling curious?
- [All] Yeah!
- Excellent!
Today on Curious Crew... (drum tapping) - Whoa.
- [Rob] The crew is all ears... - Why is this so hard?
- [Rob] As we pump up the volumes... (glass ringing) And explore the speed of sound.
Full speed ahead, you guys!
- [Announcer] Support for Curious Crew is provided by MSU Federal Credit Union.
From sweet peas to teens, MSU FCU offers you accounts that grow with children.
With financial education, gaming apps, and events, MSU FSU provides the tools and resources to make learning about finances fun and interactive.
Also by the Consumers Energy Foundation, dedicated to ensuring Michigan residents have access to world-class educational resources.
More information is available at consumersenergy.com/foundation.
Consumers Energy Foundation, supporting education and building sustainable communities in Michigan's hometowns.
And by viewers like you!
Thank you!
(upbeat rock intro music) - Hi, I'm Rob Stevenson, and this is... - [All] Curious Crew!
- [Rob] Welcome to the show, everybody.
We always like to start every episode with a couple of discrepant events.
Because discrepant events stimulate... - [All] Curiosity!
- [Rob] That's exactly right!
And I've got some fun ones for you today!
First, I wanna direct your attention to this chamber right over here.
Now, this whole thing is plastic, and there is actually a rubber seal in between the top and bottom.
The other thing that's obvious to notice is there's an alarm bell that is inside and that's actually electrically connected coming out of the system.
And I can plug it in to this battery and get that little hammer to ring, okay?
(bell ringing) Now, on the back of the system, we have a tube that's coming out, and this tube is going down to a vacuum pump that I have on the floor that I can control with a foot pedal.
So here's what I'm wondering: if I turn on the alarm bell, and then turn on the vacuum pump, what might happen?
Jacob, what do you think?
- Will the noise stop?
- Ooh, that is a really interesting prediction.
I guess the only way we're gonna know is if we try it.
So I'm gonna start this up.
(bell ringing) And here goes the vacuum.
(vacuum buzzing) Now, you might have noticed, I just pinched off the tube at the back.
What are you noticing now?
Callen, do you have an observation?
- It got a lot quieter?
- It's actually quieter.
Watch the system closely as I remove the channel locks and see what happens.
(air hissing) (bell rings louder) So we not only have some air coming flying in there, it actually gets louder.
Now, we've got another discrepant event we wanna look at right here.
And Nash has one at home, just like I have here.
I've got a tuning fork.
And on the end of the tuning fork is a string that goes all the way up and is threaded through a funnel.
The string goes over the side of the funnel and is taped there.
Now, Nash and I are gonna strike this tuning fork right on this little block and dangle it down and see what we notice.
You ready, Nash?
Let's do it.
(hits block) (hits block) So Nash, what do you notice?
- The sound is a lot clearer.
- Isn't that strange?
I'm gonna invite three of you to do a little scientific modeling, to see if you can come up with an explanation by the end of the show.
I'm gonna ask you to use your background knowledge, any information you learned throughout the show to revise your thinking, to see if you can come up with an explanation.
Who wants to try a little modeling moment?
Who would like to do that today?
Okay, how about Julia, Carmela, and Nash?
Excellent!
Now, I have one final question: What do you think we're gonna be investigating today?
Ollie, what do you think?
- Is it sound?
- Oh, excellent!
We are going to be discussing the speed of sound!
Stick around.
This sounds like we're going full speed ahead!
(upbeat hip hop music) - The string sound reminded me of talking through a string telephone, where there's a string that connects two cups, and then you talk through one cup and somebody else can hear your voice through another cup.
- Well, I've done that before.
I remember you had to keep the string really tight for it to work.
So I thought it was really cool that we could hear the sound so well.
- I was thinking about the alarm bell.
When Dr.
Rob pumped air out of the system, particles must be leaving.
But then air was rushed into the system at the end, and the sound got louder.
(upbeat hip hop music) (hands clapping together) - [Rob] We are surrounded by different sounds.
The birds singing in the trees, the neighbor's lawnmower, even the TV you're watching.
Each sound is a vibration that starts the disturbance that transfers energy and waves through particles of matter that we interpret as sound.
The particles bump into each other and transfer that pressure wave over a distance.
But the speed the sound travels is affected by the material's elasticity, what state of matter it is, its density, and even its temperature.
Sounds interesting?
(action techno music) Weren't those discrepant events interesting, you guys?
- [Both] Yeah!
- So we are gonna do a little bit more trying to figure out speed of sound.
And we're gonna start with a couple of PVC pipes.
Grab those pieces of PVC.
And I know that we've got some membranes over both the top and the bottom.
Here's what I'm gonna ask you to do: Once you look at the top membrane and very gently just press on the bottom... Oh wow, Callen.
Yeah, yours is really bulging up.
Yeah, I can see both of them bulging up!
Okay, now what I want you to do is lay a finger across the top and use that little mallet and gently tap on the bottom.
Callen, what are you noticing?
- I can feel the vibrations of it.
- Excellent, okay.
So you can definitely feel the vibrations coming through.
This is a closed system, which is interesting.
Whether we're pressing on the bottom or tapping on the bottom, we can see the effects of that on the top.
But what about if this were an open system?
What if we cut the drum in half?
Let's take a look at this over here.
I've got two drums that are independent of each other, hanging from some ring stands.
And beside one of my drums, I've got this ping pong ball suspended there.
Now, my question for you, Ollie, is what do you think is gonna happen if I strike this drum?
- I don't think that the ping pong ball's gonna move.
I think that the drum is just gonna make a sound.
- Okay, awesome.
Let's try it.
(drum tapping) - Whoa.
(Rob laughing) (drum tapping) - Surprise!
Now, that seems perplexing.
They're not attached.
And why would that still move?
Well, it all has to do with the sound waves that are traveling through the air between the drums.
Obviously, when I strike the drum, it oscillates, but then it starts colliding with the air particles, which transfers all the way to the other side.
But what I wanna do is, let's calculate how fast that speed of sound is actually going through the air.
Okay?
Now, to do that, we're gonna use some pipes.
If you look at these pipes right here, I've got a number of different colored pipes and they seem like they're empty, but are they really empty, Callen?
- No, they have air in them.
- Of course.
They have air in them.
Exactly right.
So if I strike them... (pipes tapping) they're going to produce a certain sound and that's gonna correlate with how long the pipe is.
(pipes tapping) That makes sense.
Now, we're gonna calculate this using this pipe, another empty pipe, or pipe that's filled with air.
I've got some earbuds that I've attached to the top, and I have it plugged into my computer.
I'm gonna play a tone that produces 440 hertz.
Now, to make this really interesting, I'm gonna put this down inside the water.
And when I start the tone, we're not gonna really hear very much.
So I'll start lifting it out of the water.
And when you guys can hear it, you tell me to stop.
Okay?
Here we go.
(pipe humming) - [Both] Stop.
- Okay, right there.
Now, very carefully, I'm gonna grab my tape measure.
I'm going to measure from the surface of the water up to the top of the pipe.
I've got it.
That is now one quarter of a wavelength.
If I take that number, multiply it by four, I can find the wavelength.
And if I take that number and multiply it by the frequency, what was the frequency again, Ollie?
- 440 hertz.
- Exactly, 440 hertz.
I can figure out the speed of sound that it's traveling in the air in this column.
And based upon the studio and the temperature in here, we're looking at about 20 degrees Celsius, which means the sound was traveling at 343 meters per second!
That's like taking 343 meter sticks, putting them end-to-end, and traveling that entire distance in one second!
Now that sounds amazing!
We know that sound waves in the air are longitudinal waves, and the energy makes the air particles collide back and forth.
If the air temperature were 21 degrees Celsius, or almost 70 degrees Fahrenheit, the sound wave would travel at 343.6 meters per second.
But when it's warmer, the air molecules move faster.
So the sound wave travels faster, too.
At 45 degrees Celsius, sound and air travels 358 meters per second.
While at -1 degree Celsius, it only travels 330.4 meters per second.
Speed of sound is affected by temperature!
Amazing!
(action techno music) So we've explored the speed of sound in air, but what about liquid?
So have either of you been swimming underwater and suddenly you heard something?
- [Both] Yeah.
- [Rob] Julia, gimme an example.
- When I tried talking to people while underwater.
- Doing the whole tea party, trying to talk to people.
Which is really tricky, be careful doing that!
But you can hear how the sound travels.
I've taken a stethoscope, and I've taken the stethoscope apart and replaced the end with a funnel.
Now, I filled the entire system up with water, all the way out to the earpiece here.
I'm going to strike a tuning fork and place the tines of the fork inside the funnel.
That disturbance is gonna go all the way through the tube.
And we're gonna pick up the sound with the microphone.
You guys ready for this?
It's pretty cool.
(tuning fork dings) Now, what's crazy is it seems to stop as soon as we put the times in the water.
It splashes like mad and it seems to stop.
But that sound wave has already gone all the way through the system and got picked up.
Amazing!
Now, Jacob, you have something in your hand there.
What have you got?
- I have this glass tube that's filled with water and it has bells inside.
- And Julia, you've got one with a bottle, I think?
Is that right?
- Yeah.
- Okay.
Now, I'm gonna ask you guys to hold those near your ears and shake 'em, okay?
Just give that a shake.
(rattling) Julia, what do you notice?
Can you hear it?
- Yeah, I can hear the bells.
- Yeah, the sound comes through really well.
It's going through the water through the side of the container and actually through the air.
Now, I wanna show this with some larger bells and using my big container of water here.
So I've got some holiday bells.
Nice sound.
(bells ringing) We're gonna put it inside.
(muffled jingling) Now, although it's muffled, I can still hear it.
I wanna show this with a bicycle bell as well.
Now, if you've ever used a bicycle bell before, you know that it's got a really nice little ring to it.
There it is.
(bicycle bell rings) And I'm gonna put this underneath and give it a little... (muffled clicking) Now, we don't get quite the resonance, but we can hear the sound.
Now, here's something I want you to think about.
It might surprise you to know that the speed of sound is actually going faster through water than it is through the air.
We know that in air, at about 20 degrees Celsius, it's going at 343 meters per second.
But in liquids, it can travel 1,481 meters per second!
That is ridiculously fast!
So Jacob, why might the sound travel faster through a liquid than through the air?
- Is it because the molecules are closer together?
- That is exactly why!
If the particles are closer together, they bump into each other and the disturbance moves even faster.
Now, going through fresh water at 1,481 meters per second, that's really fast.
But if we did it through salt water and I put salt water in the system, it would go even faster.
And that's because salt water has a higher density.
So the next time you guys are swimming, you're underwater, and you hear some sounds, you can think, "Whoa, that sound is going really fast."
Have you ever been swimming in a lake or pool, and heard sounds underwater?
The molecules and liquids are closer together than those in gases.
So they can bump into each other much more quickly than the further-spaced particles found in the air.
That means the sound waves can transfer energy through the liquid particles much more quickly.
That also means the sound you heard could've been traveling at 1,480 meters per second!
That sure is some speedy sound!
(fanfare) So have you guys ever heard the expression "Talking to hear the sound of one's own voice"?
- [All] Yeah.
- So we're gonna make that expression a reality today with your Stem Challenge.
In fact, I'm challenging you to make what's called an auditory loop.
And this is a device that can quickly and efficiently move the sound of your voice to your own ear.
Oh wow, I can hear myself!
So are you guys ready to start your build?
- [All] Yeah!
- All right, let's do it!
- So Dr.
Rob has us making these auditory loops.
Put the pipe cleaner on the funnel... - Mine has a funnel in which you talk into, and then the audio comes out into these two tubes that you stick in your ears, and then you can hear yourself.
Oh, oh.
- I used a stethoscope and a funnel.
Oh, and a pipe cleaner.
Can't forget the pipe cleaner.
- The biggest challenge for me was when I had to tape the headband onto the tubes that you put into your ear, because they kept falling and slipping.
- Just go over my head.
Why is this so hard?
When I finally got it to work, I could hear myself.
When I spoken into it, it was really, really loud in my ears.
Okay.
Oh wow, that is weird.
- La la la la la... - Let's take a look at what you've got there.
Oh, yes.
You can even do a little test.
One, two, three... - Hello?
- [Rob] Testing one, two, three.
So Callen, what are you noticing?
- That my voice is really clear and it's really loud.
- It's really loud.
That column of air that's carrying your voice is pretty small.
The sound travels really efficiently, and you're probably noticing it's picking up even the smallest of sounds.
So talking in a quiet voice would be smart.
Try making your own auditory loop and let us know what you did!
We have seen that sound can travel through different materials, and travel quickly.
But what happens if the object making the sound moves even faster through the air than the sound wave itself?
That object is traveling at supersonic speed, and would create a loud noise called a sonic boom.
This is because a high-energy shock wave in the air is produced that visibly squishes the air particles and sounds like a large explosion.
Chuck Yeager was the first pilot to break the speed of sound in the Bell X-1 in 1947.
But there are natural examples, too.
Each time lightning strikes, the thunder you hear is another example of a sonic boom.
(thunder strikes) Wow!
(active techno music) So we've been exploring the speed of sound in air... in water...
But what about solids?
Can you guys think of examples where you've actually felt sound through a solid?
Carmela, can you give an example?
- Sometimes if there's music playing on a speaker and you put your hand on it, you can feel the vibrations from the speaker.
- Oh, that's a great example!
Okay, we're gonna explore some more.
You ready?
Now, I know you guys have some tuning forks standing by, and I'm gonna ask you to do something with your tuning fork.
I'd like you to strike it on your block and then move it away from your ear.
And let me know what you notice.
(Nash hits block) (dings) Nash, what do you notice when you do that?
- The sound gets quieter.
- It gets a lot quieter, doesn't it?
Okay, now what I'm gonna ask you to do is holding an aluminum ruler up to your ear, I'm gonna ask you to strike it again, and this time, put the base of the tuning fork right on the end of the ruler.
Go ahead and try it.
(strikes block) (faint ringing) Carmela, what do you notice?
- Even though the tuning fork is really far, you can still hear the sound clearly.
- That is excellent!
When we did it in the air like this, we couldn't hear it anymore.
But going through the solid ruler, you can hear it really, really well.
Now, that's really an interesting phenomenon, but we have particles that are a lot closer together.
And if they're closer together, they bump into each other faster and can pass that disturbance along, right?
Let's look at this another way.
I've got a couple glasses here, and I'm gonna dip my finger in some vinegar.
And I'm gonna see if I can get this glass- Oh yeah, that's a thing.
(glass ringing) Now, we can hear that sound going through the air.
And we figured out it's about 343 meters per second here in the studio.
But we also can see the effect of the water inside, and that vibration's traveling even faster.
But let's see if we can find evidence of the sound going through the glass.
I'm gonna move these glasses closer together and see if we can get a reaction in the other glass.
(both glasses vibrating, ringing) So you can hear that buzz going through.
Now, we know in the air, it's traveling at 343 meters per second.
It's going faster still in the water, but in glass, it can travel 4,540 meters per second!
That is really, really fast!
Let's look at this another way.
I've got a model over here with two rows of blocks, okay?
And you'll notice the back row, the blocks are closer together.
This is actually going to represent particles in a solid.
The front row are going to represent particles in a liquid.
So they're spaced apart.
Now, if I start a disturbance and start tipping them over, I wonder which one is going to have an impact on the balloon on that end.
But I've got some tacks on the top of the last block.
So Nash, which balloon do you think might pop first?
The green one for the solids, or the blue one for the liquids?
- I think it's gonna be the green one.
- [Rob] He's going with the green one.
Okay, so I'm gonna carefully slide this out.
Try not to cause a disturbance too early and then place this down below.
Let's see if Nash's prediction is right.
Full speed ahead, you guys!
(blocks falling) (balloon pops) Oh my gosh!
Okay, so Nash, first of all, your prediction was spot on!
The green one did pop first.
But look at this discrepant event.
The blue one didn't pop!
Even though the pin grazed the top, we ended up having a little bit of an elastic bounce right off of the balloon, which was awesome, and the angle of the pin just did not penetrate.
Obviously we have the particles closer together.
So the sound is going to travel faster in this model than in the other.
Now, if you really want to think about it, if we would've substituted aluminum as our model, aluminum is a surefire winner.
Talk about pedal to the metal!
(upbeat orchestra music) - Are you curious about careers in science?
Hi, I'm Janellyn, and with me today is Kristen Lueck.
Kristen, tell me where you are and what you do.
- [Kristen] I'm in New York City, and I'm a sonic strategist working in sonic branding.
- What is sonic branding?
- [Kristen] Sonic branding is using music and sound to tell brand stories.
Music and sound play powerfully on our emotions, just as scent does.
Sound impacts our experiences.
So using strategic insights, we can craft original sound and music for our clients.
- How does sound affect our brains?
- We're wired for it.
Music and sound carry messages and stimulate emotions, just like words and visuals do.
But even more so, because music is so elementally human, the sound of a brand is an important brand asset, just like a visual logo.
(cymbal hitting) - Hearing about sonic branding from Kristen Lueck showed me how sound affects our lives.
Explore your possibilities!
(upbeat orchestra music) And now, back to Curious Crew!
(gentle techno music) (upbeat hip hop music) - We know that the sound can travel through the string, just like it traveled through the solid ruler.
- Or even if we place the tuning fork against our heads.
- Right.
So the sound can move quickly through particles in the string more efficiently than it can move through the air.
- I agree.
And with the alarm, there are less air particles in the container.
- That's right.
So the sound travels less efficiently when there are fewer particles.
We've seen during all of our investigations that the sound needs a medium to travel through.
- So have you guys had fun investigating speed of sound today?
- [All] Yeah!
- Excellent!
So now, I know you've been thinking a lot about these phenomena from the beginning of the show.
So it's time to circle back to see if we can explain them.
So what have we figured out about the string sound, Nash?
- We know that it's easier to hear the sound through the string because sound travels easier through most solids than it does through the air.
This is because the particles are closer together, allowing the sound to travel more quickly.
- Excellent!
So we know the sound can travel through the string, it can go through the funnel, and it's actually easier for us to hear, just like you commented on at the beginning.
Think about it this way: If we've got particles that are closer together, the energy can move through the system much more quickly than if the particles were spaced apart.
The other thing to keep in mind is the elasticity of the material.
And what I mean by that is how quickly will the object go back to its original shape?
This is all dependent on the bonds between the particles.
If there's really strong bonds, they get right back and the disturbance goes through really efficiently.
The other thing to think about is the density.
If we've got particles that are super, super massive, the energy moves a lot more slowly through that.
But for the most part, we're looking at much faster rates through solids than through either liquids or gases.
Good job, you guys.
Now, how about the silent alarm, Julia?
What have you guys figured out about that?
- We know that sound waves transfer energy through the particles in solids, liquids, and gases.
And we also know that the closer the particles are together, the faster the energy moves.
- Right.
And if you pump air out, there are fewer particles that are spaced even farther apart, which affects the sound.
- Great thinking, everybody!
So we know that in air at about 20 degrees Celsius, sound can travel at about 343 meters per second, but it still needs particles to move through, right?
If there's no particles there, how can we move a disturbance through there?
So here's a wonder I have for you, Carmela: What would've happened if we could have eliminated or removed almost all of the air particles inside?
- It would be silent, just like how there's no sound in space.
- [Rob] Great job, everybody!
So we've seen how the speed of sound is affected by temperature, elasticity, density, and even the state of matter.
Well, looks like we're up to speed!
And that sounds pretty terrific to me.
So remember, my friends... - [All] Stay curious!
- And keep experimenting!
Get your curiosity guide and see more programs at wkar.org.
- [Announcer] Support for Curious Crew is provided by MSU Federal Credit Union.
From sweet peas to teens, MSU FCU offers you accounts that grow with children.
With financial education, gaming apps and events, MSU FSU provides the tools and resources to make learning about finances fun and interactive.
Also by the Consumers Energy Foundation, dedicated to ensuring Michigan residents have access to world-class educational resources.
More information is available at consumersenergy.com/foundation.
Consumers Energy Foundation, supporting education and building sustainable communities in Michigan's hometowns.
And by viewers like you!
Thank you!
- Pump it up, pump it up, pump it up!
We got this!
- Investigation One.
(claps) - That was a great slate.
Judges say "pshh"!
(Rob laughs) And then we'll see if we can start.
(blocks falling) (Rob laughs) And that's why we have an extra balloon!
(blocks falling) (balloon pops) Ohh, yes!
The un-poppable.
Look at that!
The pin is right in it!
(soft piano music)
Support for PBS provided by:
Curious Crew is a local public television program presented by WKAR
Support for Curious Crew Season 10 is provided by
MSU Federal Credit Union
Consumers Energy Foundation